Solar flares are powerful bursts of electromagnetic radiation originating from the Sun’s surface, caused by the sudden release of magnetic energy stored in active regions near sunspots. These dynamic and energetic phenomena are among the most dramatic expressions of solar activity, often accompanied by intense brightening in the solar atmosphere. The energy released during a solar flare can range from the equivalent of millions to billions of nuclear bombs, making them a significant subject of study for scientists seeking to understand the Sun’s influence on the solar system.
The Sun’s magnetic field is the driving force behind solar flares. In regions of intense magnetic activity, magnetic field lines can become twisted and stressed. When these lines realign or snap, immense energy is unleashed, producing flares that emit radiation across the electromagnetic spectrum, including X-rays, ultraviolet light, and radio waves. Solar flares are classified based on their X-ray intensity into categories A, B, C, M, and X, with X-class flares being the most powerful. While the radiation from flares travels at the speed of light and reaches Earth in about eight minutes, their effects on our planet are far-reaching and varied.
Solar flares can disrupt Earth’s technological systems and atmospheric conditions. The high-energy radiation ionizes Earth’s upper atmosphere, affecting communication systems such as shortwave radio and GPS. Additionally, satellites, power grids, and even astronauts in space can face challenges from the increased radiation levels. Despite their potentially disruptive effects, solar flares also serve as a reminder of the interconnectedness of the Sun and Earth, driving phenomena like auroras and influencing space weather. Understanding solar flares is essential for predicting and mitigating their impacts, ensuring the continued resilience of modern technology and infrastructure.
The origin of solar flares
To understand the origin of solar flares, it is essential to explore the Sun’s structure, its magnetic field dynamics, and the mechanisms that trigger these explosive events.
The Sun’s magnetic field: a fundamental driver
The Sun’s magnetic field is the primary force behind the formation of solar flares. Generated by a process known as the solar dynamo, this magnetic field arises from the movement of plasma within the Sun’s interior. The Sun is composed mainly of ionized gas, or plasma, which moves in complex patterns due to convection, rotation, and differential motion between its equator and poles. These movements twist and tangle magnetic field lines, creating regions of intense magnetic stress and energy storage.
Sunspots, dark patches on the Sun’s surface, are visible indicators of magnetic activity. These regions have much stronger magnetic fields than the surrounding areas and are cooler due to suppressed convection. Solar flares often originate from active regions around sunspots where magnetic field lines are highly concentrated and twisted. The magnetic energy in these regions can build up over time, leading to the conditions necessary for a solar flare.
Magnetic reconnection: the spark of solar flares
The immediate trigger for a solar flare is a process known as magnetic reconnection. This occurs when oppositely directed magnetic field lines come into close contact and realign, releasing vast amounts of stored magnetic energy in the process. Magnetic reconnection is facilitated by the highly conductive plasma in the Sun’s atmosphere, which allows magnetic fields to interact dynamically.
In an active region, the magnetic field can become so stressed and tangled that it destabilizes. When reconnection happens, the energy previously stored in the twisted magnetic field is converted into heat, kinetic energy, and accelerated particles. This energy is then released as radiation across the electromagnetic spectrum, from X-rays and gamma rays to visible and radio waves. The intensity of a solar flare depends on the amount of energy stored and released during this reconnection event.
The role of the solar atmosphere
The Sun’s atmosphere, or corona, plays a crucial role in the formation and propagation of solar flares. The corona, characterized by its extreme temperatures and low density, is threaded with magnetic field lines that connect to the Sun’s surface. When magnetic reconnection occurs, it heats the corona to tens of millions of degrees Kelvin, producing the intense X-rays and ultraviolet radiation that are hallmarks of solar flares.
Additionally, the process accelerates charged particles such as electrons and protons to near-light speeds, which then interact with the solar atmosphere to generate high-energy emissions. These accelerated particles also travel along magnetic field lines, sometimes escaping the Sun and contributing to solar energetic particle (SEP) events, which can impact Earth’s space environment. The complex interplay between the Sun’s magnetic field and its atmospheric plasma makes the corona an essential part of the solar flare formation process.
Solar activity cycles and flare frequency
The Sun’s activity varies over an approximately 11-year solar cycle, during which the frequency and intensity of solar flares change. At the solar maximum, when the Sun’s magnetic field is most active and sunspots are numerous, solar flares occur more frequently. Conversely, during the solar minimum, when the Sun’s magnetic field is less dynamic, flares are relatively rare. This cyclic nature of solar activity reflects the underlying dynamo processes and the periodic regeneration of the Sun’s magnetic field.
The largest solar flares, known as X-class flares, are most likely during the solar maximum. These events have the potential to produce significant space weather effects, such as geomagnetic storms and disruptions to communication systems on Earth. Understanding the solar cycle and its influence on flare activity is crucial for predicting these events and mitigating their impact on modern technology.
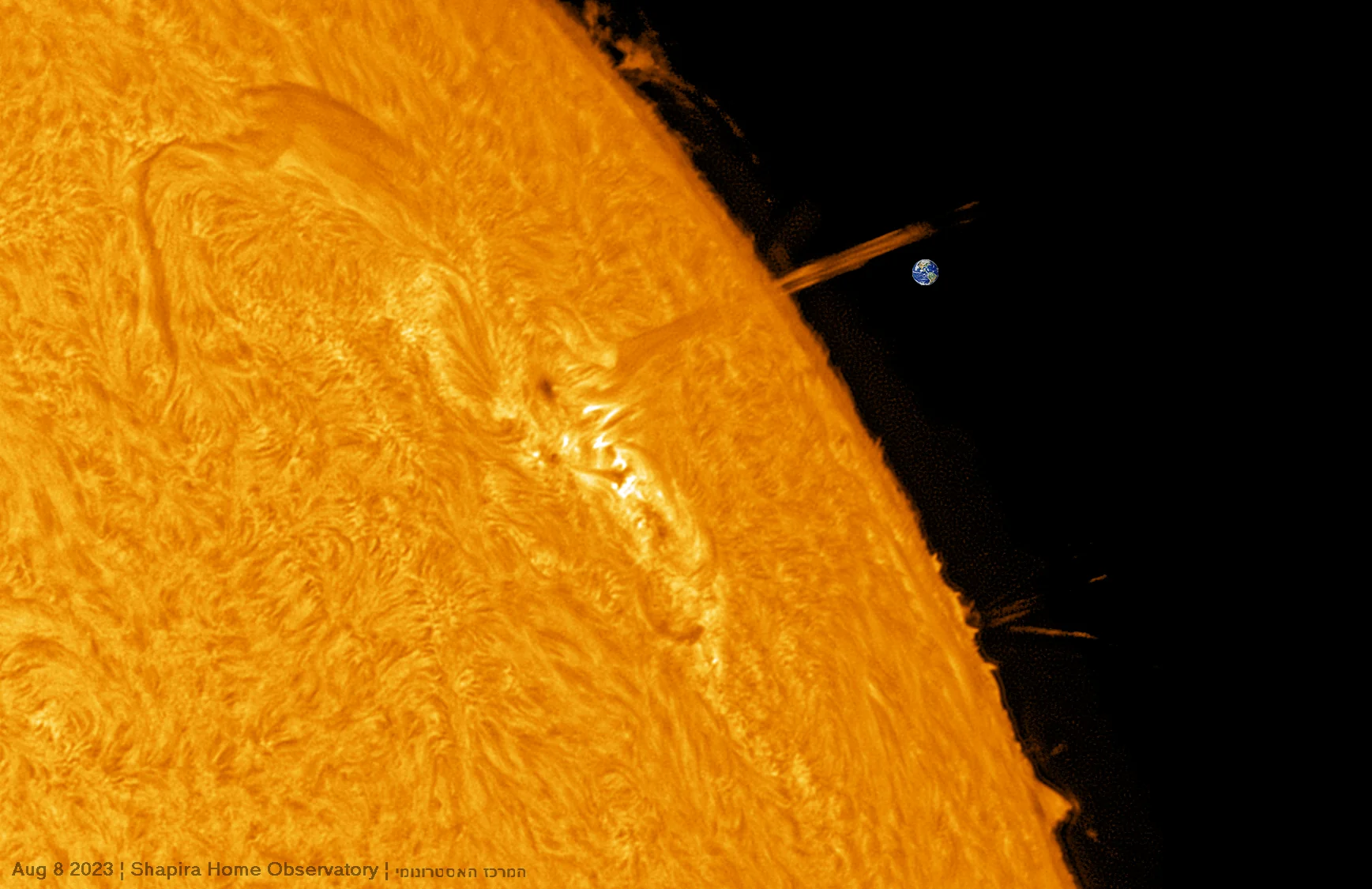
Effects of solar flares on earth
Solar flares, explosive bursts of energy from the Sun’s surface, have profound effects on Earth and its surrounding space environment. These intense eruptions, caused by the sudden release of magnetic energy in the Sun’s atmosphere, emit radiation across the electromagnetic spectrum. While Earth’s magnetic field provides some protection from these phenomena, the consequences of solar flares can still extend to our planet’s atmosphere, technology, and infrastructure. Understanding these effects is essential for mitigating their impact on modern society.
Impact on Earth’s atmosphere and ionosphere
The ionosphere, a layer of Earth’s atmosphere rich in charged particles, is directly affected by the radiation from solar flares. High-energy X-rays and ultraviolet (UV) radiation ionize the atoms and molecules in the ionosphere, causing disturbances in its density and composition. These changes can lead to disruptions in high-frequency (HF) radio communications, which rely on the ionosphere to propagate signals over long distances. This phenomenon, known as a radio blackout, can be particularly problematic for aviation, maritime operations, and emergency services that depend on HF radio for communication.
In addition to radio disruptions, solar flares can enhance the conductivity of the ionosphere, altering its interaction with Earth’s magnetic field. This can intensify geomagnetic currents, which are linked to other space weather phenomena such as auroras. The sudden influx of energy from a solar flare can also heat the upper atmosphere, causing it to expand. This expansion increases drag on low-Earth orbit satellites, potentially altering their trajectories and reducing their operational lifespans.
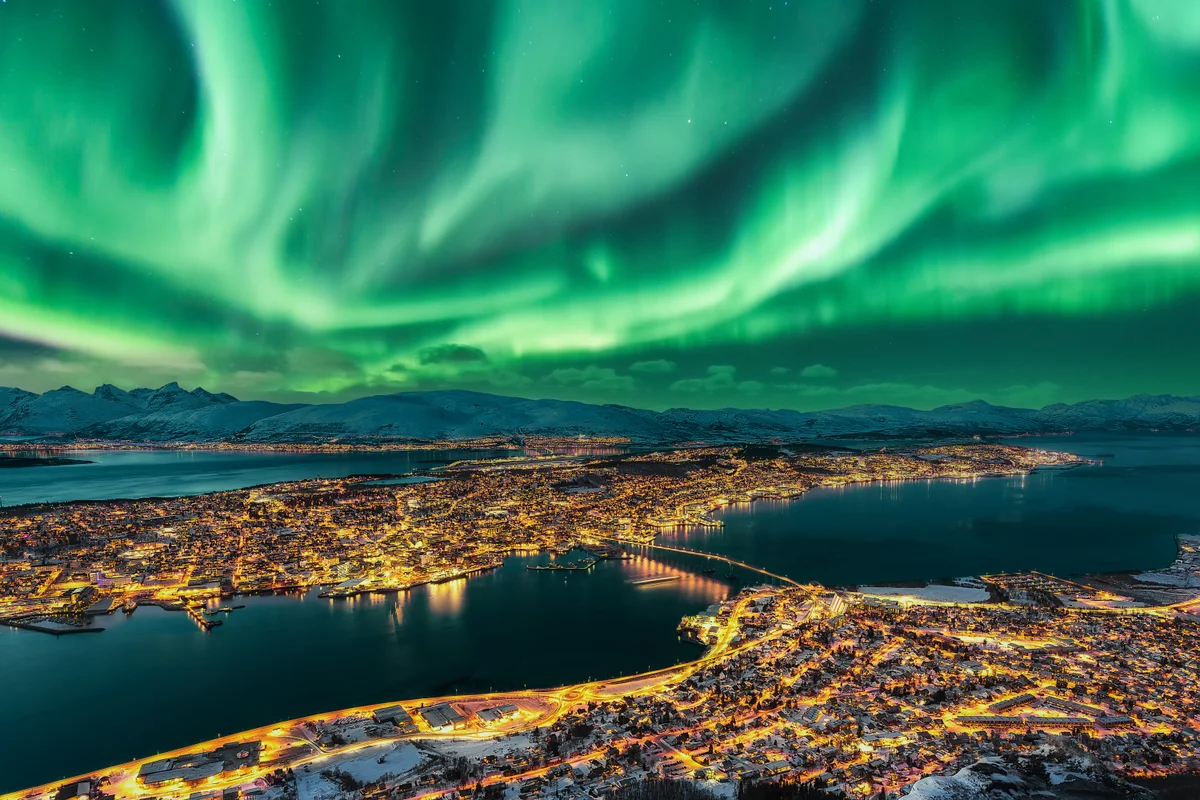
Effects on communication and navigation systems
Modern communication and navigation systems are particularly vulnerable to the electromagnetic radiation and energetic particles produced by solar flares. Global Positioning System (GPS) signals, which depend on the stability of the ionosphere, can become unreliable during solar flare events. Disruptions in GPS signals can affect various applications, from navigation in aviation and shipping to precision farming and financial transactions. Satellite-based communication systems are also at risk, as the intense radiation can interfere with signal transmission and reception.
Solar flares can also generate strong radio bursts that directly affect ground-based technologies. These bursts can overwhelm communication frequencies, causing temporary outages. The cumulative effect of these disruptions highlights the importance of developing resilient communication and navigation systems capable of withstanding solar flare impacts. Enhanced monitoring and predictive tools are essential for providing advance warnings and minimizing the operational challenges posed by these events.
Implications for power grids and infrastructure
Although more closely associated with coronal mass ejections (CMEs), solar flares can still indirectly affect power grids through their influence on the ionosphere and geomagnetic environment. Intense solar flares can initiate geomagnetically induced currents (GICs), which can flow through power lines and transformers. These currents pose a risk of overheating and damaging electrical infrastructure, leading to power outages on regional or even global scales. Historical events, such as the 1989 geomagnetic storm that caused a widespread blackout in Quebec, Canada, illustrate the potential vulnerability of power grids to space weather phenomena.
Efforts to mitigate these risks include the implementation of better forecasting models and protective measures, such as grid-hardening technologies. Operators are increasingly relying on space weather alerts to prepare for potential disruptions. By understanding the connection between solar flares and geomagnetic disturbances, scientists and engineers aim to build more resilient energy systems capable of withstanding solar-induced challenges.
Risks to human health and space operations
The energetic particles released during solar flares pose a significant risk to human health, particularly for astronauts and airline passengers on high-altitude polar routes. In space, where Earth’s magnetic field offers limited protection, astronauts are exposed to higher levels of radiation during solar flare events. This radiation can damage biological tissues, increasing the risk of cancer and other health issues. Space agencies have protocols to protect crew members during intense solar activity, such as relocating them to shielded areas within spacecraft.
Airline passengers and crew on polar flights are also exposed to increased radiation levels during solar flare events, as the Earth’s magnetic field is weaker near the poles. Airlines may reroute flights to lower latitudes during periods of heightened solar activity to minimize exposure. Additionally, the radiation from solar flares can disrupt onboard communication and navigation systems, adding operational challenges to high-altitude flights.
Broader impacts on society and technology
The broader implications of solar flares extend beyond immediate technological disruptions. As society becomes increasingly reliant on satellite-based systems for communication, navigation, and weather forecasting, the need for robust space weather monitoring becomes critical. Space weather forecasters, using advanced observational tools like the Solar Dynamics Observatory (SDO) and the Geostationary Operational Environmental Satellite (GOES) series, provide essential data to predict and mitigate the effects of solar flares.
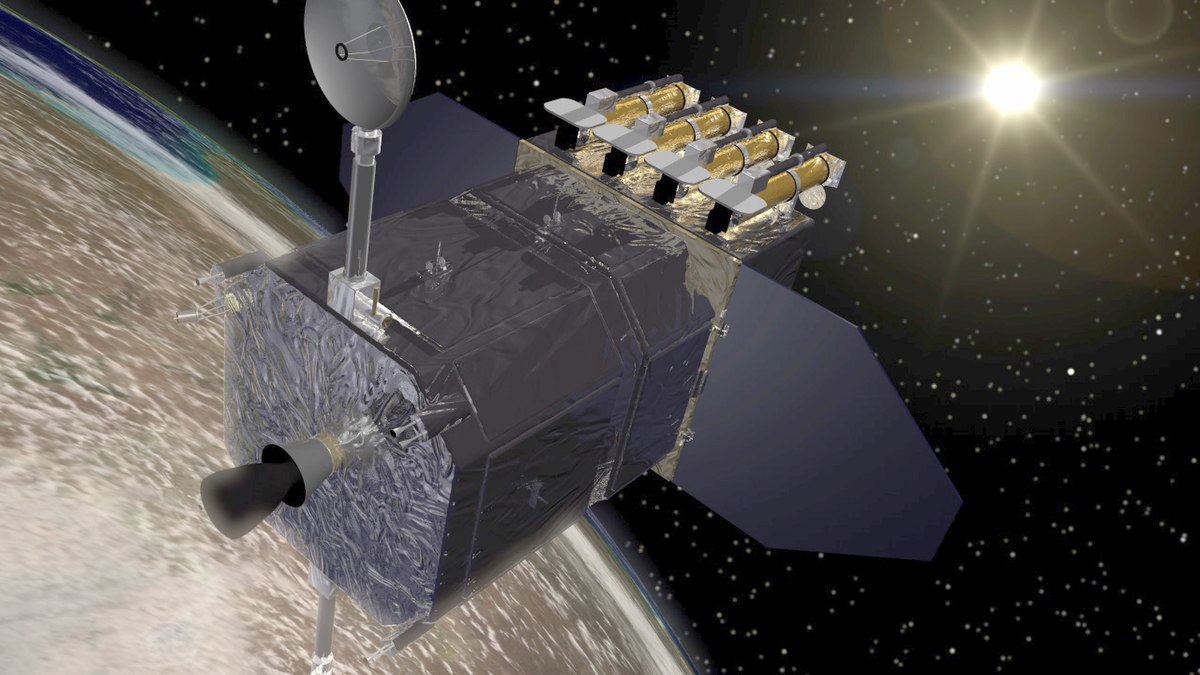
In addition to technological concerns, solar flares also have cultural and scientific significance. They drive auroral displays, creating awe-inspiring natural phenomena that connect people to the Sun’s dynamic processes. On a scientific level, studying solar flares provides valuable insights into magnetic reconnection, particle acceleration, and plasma physics, deepening our understanding of fundamental astrophysical principles. By improving our knowledge of solar flares and their impacts, we can better protect our infrastructure and harness the Sun’s power for the benefit of humanity.
Solar flare monitoring and prediction
Solar flares, powerful bursts of electromagnetic radiation from the Sun, pose significant challenges to modern technology and infrastructure. These energetic events can disrupt communication systems, navigation networks, and power grids while posing risks to astronauts and high-altitude flights. Accurate monitoring and prediction of solar flares are crucial for mitigating their impacts. Advanced observation techniques, predictive modeling, and international collaboration are key components of solar flare monitoring and forecasting efforts.
Tools and techniques for solar flare monitoring
The monitoring of solar flares relies on a combination of ground-based and space-based observatories equipped with advanced instruments. Spacecraft such as NASA’s Solar Dynamics Observatory (SDO), the European Space Agency’s Solar and Heliospheric Observatory (SOHO), and NOAA’s Geostationary Operational Environmental Satellites (GOES) provide real-time data on solar activity. These satellites use instruments like extreme ultraviolet (EUV) imagers, X-ray sensors, and magnetographs to capture detailed images and measurements of the Sun’s surface and atmosphere.
Ground-based observatories also contribute to solar monitoring by tracking sunspots and magnetic field variations. Radio telescopes detect bursts of radio waves associated with solar flares, while optical telescopes observe changes in solar granulation and magnetic field structures. Together, these tools offer a comprehensive view of solar activity, enabling scientists to identify active regions, monitor magnetic field evolution, and detect early signs of flare formation.
Predictive models and forecasting techniques
Predicting solar flares involves the use of advanced computational models that analyze the Sun’s magnetic field dynamics and plasma behavior. One of the primary methods is the analysis of sunspots and active regions, where flares are most likely to occur. By studying the complexity and evolution of magnetic field lines, scientists can estimate the likelihood of magnetic reconnection events that trigger flares.
Machine learning and artificial intelligence (AI) are increasingly being integrated into solar flare prediction. These technologies analyze vast datasets from solar observations to identify patterns and correlations that precede flare events. For example, algorithms can track changes in magnetic field configurations and plasma flows to provide probabilistic forecasts of flare occurrence. Predictive models also incorporate data from space weather simulations, which account for interactions between the Sun’s magnetic field and the solar atmosphere.
Real-time alerts and early warning systems
Real-time monitoring systems play a critical role in providing early warnings of solar flares. Space weather agencies, such as NOAA’s Space Weather Prediction Center (SWPC) and the UK Met Office, issue alerts based on solar observations and predictive models. These alerts categorize solar flares by intensity, using the standard classification system (A, B, C, M, and X classes), and indicate potential impacts on Earth.
Early warning systems are essential for protecting vulnerable technologies and infrastructure. For instance, power grid operators can implement protective measures, such as reducing load or disconnecting critical components, to minimize the risk of damage from geomagnetically induced currents (GICs). Airlines may reroute flights to avoid polar regions during periods of heightened solar activity, reducing radiation exposure for passengers and crew. Satellite operators can place spacecraft in safe modes to prevent damage to sensitive electronics and communication systems.
Challenges and limitations in solar flare prediction
Despite significant advancements, predicting solar flares remains a complex and challenging task. One of the primary difficulties lies in the chaotic nature of the Sun’s magnetic field, which makes it difficult to accurately model its behavior. While scientists can identify regions with a high probability of flare activity, pinpointing the timing, intensity, and exact location of a flare is still an area of active research.
Another challenge is the limited lifespan and spatial coverage of observational satellites. Space-based instruments are subject to wear and tear from the harsh space environment, and gaps in coverage can occur during satellite transitions or malfunctions. Additionally, while ground-based observatories complement space-based observations, their effectiveness is limited by weather conditions and the inability to observe the Sun continuously.
The future of solar flare monitoring and prediction
The future of solar flare monitoring and prediction lies in the development of next-generation observational technologies and enhanced modeling capabilities. Missions like NASA’s Parker Solar Probe and the European Space Agency’s Solar Orbiter are providing unprecedented insights into the Sun’s magnetic field and plasma dynamics by venturing closer to the Sun than ever before. These missions aim to refine our understanding of the processes that drive solar flares, contributing to more accurate predictive models.
International collaboration is also essential for improving solar flare forecasting. Initiatives like the International Space Weather Initiative (ISWI) promote the sharing of data and resources among space agencies and research institutions worldwide. Advances in AI and machine learning will further enhance prediction accuracy by enabling real-time analysis of massive datasets. These tools will allow scientists to develop adaptive models that respond dynamically to changing solar conditions, improving the reliability of forecasts.
Interesting facts about solar flares
Solar flares are not unique to the Sun; similar phenomena have been observed on other stars, known as stellar flares. These flares can be much more energetic than those on the Sun, providing insights into magnetic processes across different types of stars. Studying stellar flares also helps scientists understand how solar-like activity might affect exoplanets and their potential habitability.
Another intriguing fact is that solar flares are often accompanied by coronal mass ejections (CMEs), massive eruptions of plasma and magnetic fields from the Sun’s corona. While flares release radiation that reaches Earth in minutes, CMEs take hours to days to arrive and can cause geomagnetic storms that disrupt power grids and communication networks.
Finally, the study of solar flares dates back to 1859 when the first recorded flare was observed by British astronomer Richard Carrington. This flare, later associated with a major geomagnetic storm known as the Carrington Event, demonstrated the profound impact of solar activity on Earth. Today, continued research and monitoring help humanity prepare for future solar events and their potential challenges.
In conclusion, monitoring and predicting solar flares are vital for safeguarding technology and infrastructure in an increasingly interconnected world. While challenges remain, advancements in observation techniques, predictive modeling, and international collaboration are paving the way for more effective space weather forecasting. By investing in these capabilities, we can better prepare for the impacts of solar flares and ensure the resilience of modern society against the Sun’s dynamic behavior.